Part 1: Applications and Geological Significance
This is the first part in a two-part series about lithium – so get excited! In this first segment we provide an overview of where lithium comes from, how we use it and why it’s important, and of course its significance in answering geological questions.
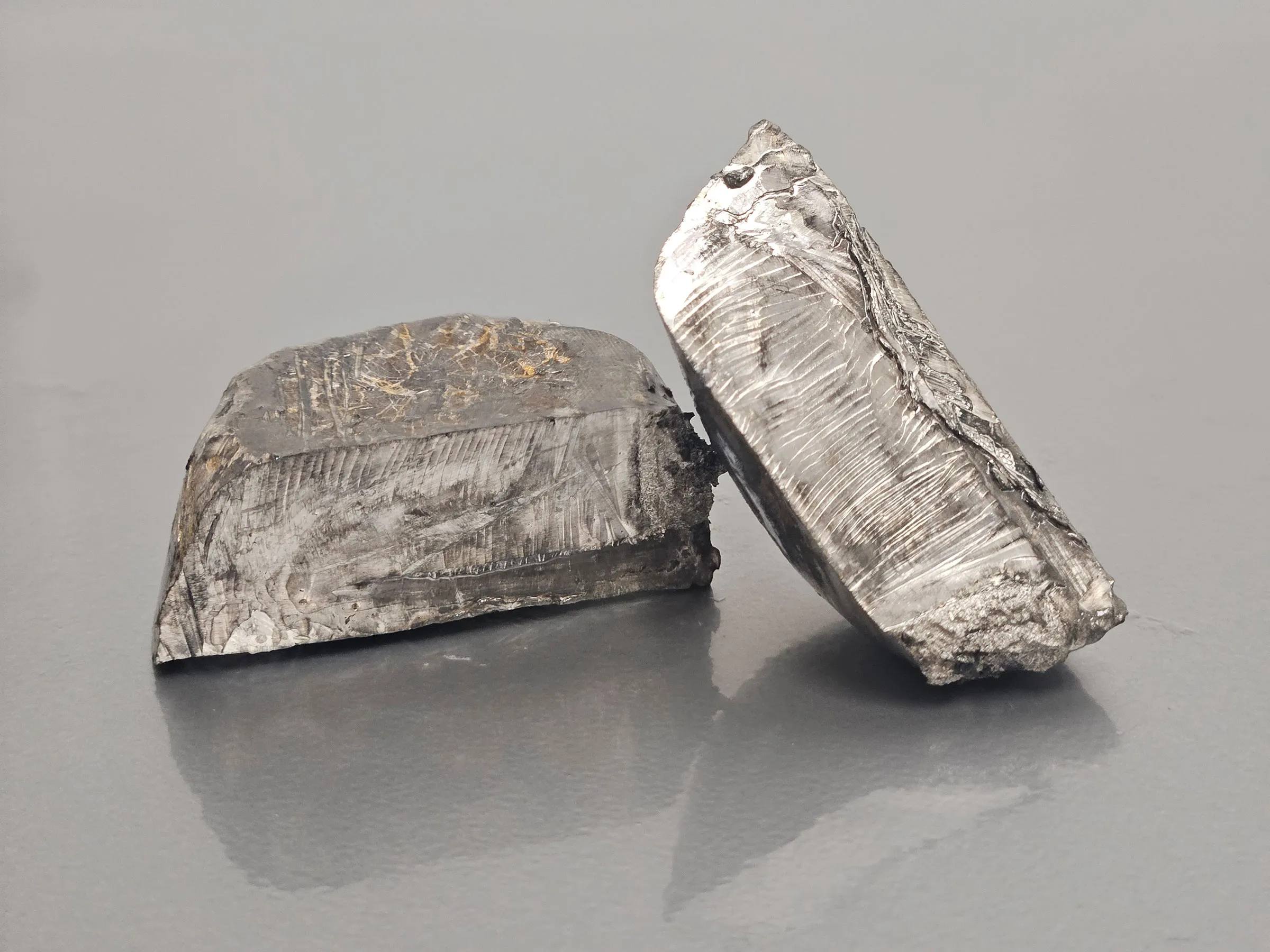
What is Lithium?
To start off, lithium is an element, specifically a metal found on the upper left-hand side of the periodic table. Lithium gets its name from the Greek word for stone “lithos” because it is present in some amount in almost all rocks. Lithium has an atomic number of 3, meaning it one of the lightest elements and the metal with the lowest density… it also readily reacts with water partly due to the extra valence electron in its outer shell (cool, right?!).
Why have I heard so much about lithium and critical minerals recently?
So now that we’ve refreshed a bit of the basics on lithium and it’s chemistry… you may be wondering what makes this element so important. You may have seen articles or heard discussions about it on the news recently! In a nutshell, this is in response to climate change in a global effort to transition away from fossil fuels… but the shift requires both new energy sources and ways to store that energy. This is where critical minerals take the stage.
In the United States, a “critical mineral” is defined as any mineral, element, substance, or material designated as ‘critical’… where ‘critical’ is a function of supply risk and overall importance to energy (US Energy Act of 2020). Thus, an increase in demand for new energy sources and storage mechanisms has directly increased the global demand for critical minerals. Now circling back to the point of this blog post, lithium is the critical mineral that ranks highest in criticality over the next 10 years!
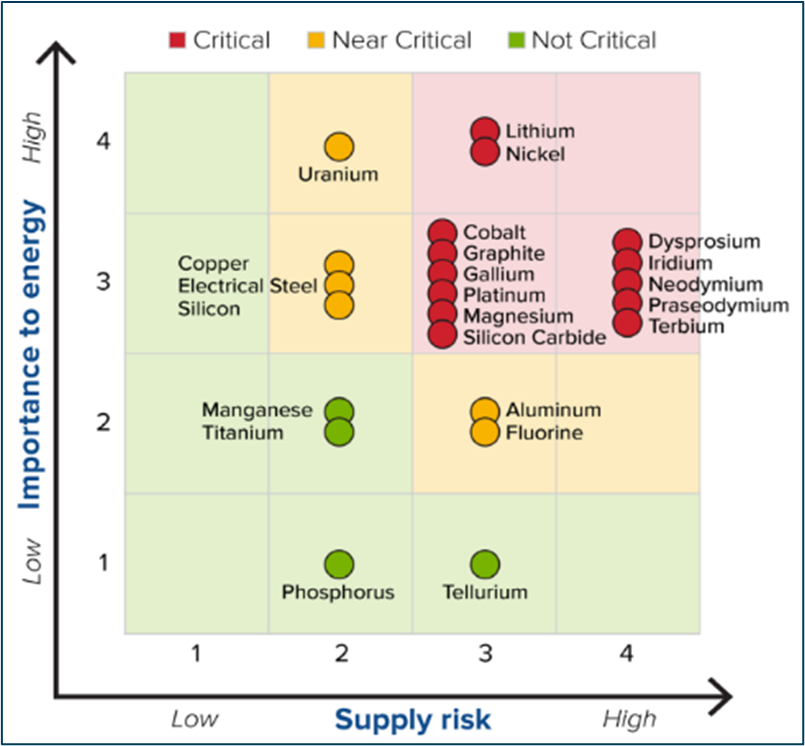
You’ve probably heard about lithium being a main component in batteries, and that’s true. But more broadly, lithium is an essential ingredient for solar panels, energy storage devices, and major components of electric vehicles. These applications are critical in the development of carbon-neutral energy sources, and the rapid transition to green technology places stress on the identification and extraction of critical mineral resources required for both energy generation and grid storage.
Did you know: While the term ‘critical minerals’ would make you assume the commodity of interest is a mineral – most critical minerals are not minerals… but often just elements!
In addition to energy storage, you may have heard references of lithium in context to medicine – quite a versatile element! In this application, lithium serves as a mood stabilizer in the treatment of people with bipolar disorder. There may be additional used for other conditions when the typical treatments are not effective, including depression, schizophrenia, and some psychiatric disorders.
The geological recipe: where do we get lithium?
So, we know we need more lithium to help fuel the ‘green’ energy transition… but where do we find this metal? The known existing resources for many critical minerals are concentrated in only a few places around the world, and the extraction processes often require large volumes of water… and the extraction can be an environmentally invasive procedure (IEA, 2021). Therefore, lithium mining is a controversial process at best, and the politics involved are beyond the scope of this blog.
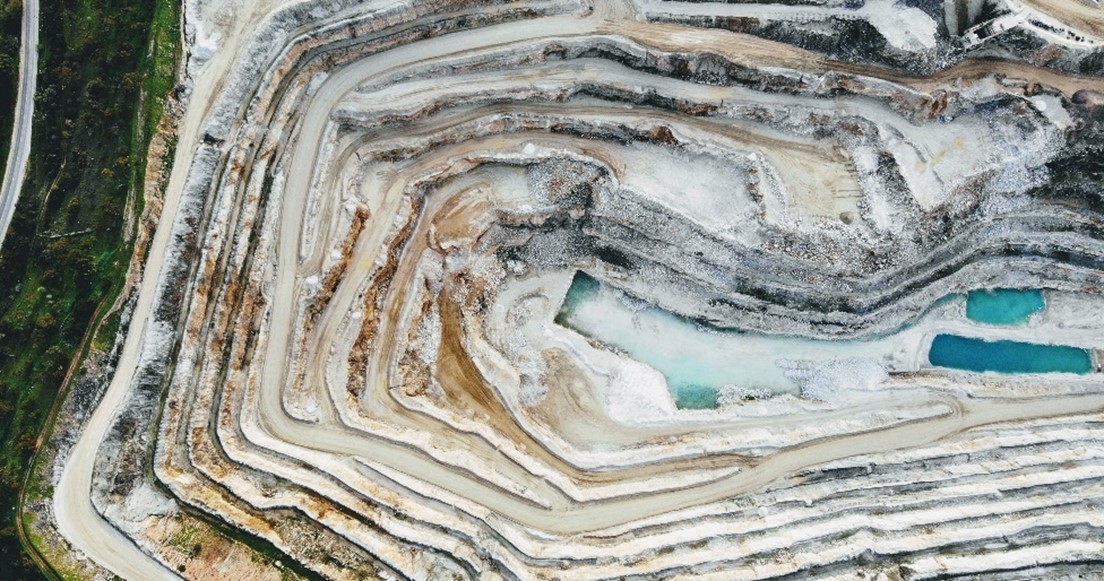
Despite their societal importance, the exact recipe of geologic processes that concentrate lithium (and many critical minerals) to concentrations that are economically viable are not fully understood. This is partly due to the complicated geologic processes involved in their formation, and because we only recently recognized the importance of these minerals’ deposits.
All that said, there are three main geologic flavors (or styles) of lithium resources which are differentiated by their geologic environment… and one of these styles was just recently recognized as economically significant (pretty wild, right?)!
These geologic flavors are listed below and color coded to match the map (figure below):

While these three styles exist around the world, they vary in how much lithium is present and what material it is found in! While there are large numbers of lithium recourses in hard rock material (i.e. pegmatites) which are economically viable, these resources tend to be small in overall amount of lithium. Some lithium deposits aren’t found in hard rock material, instead they are in evaporitic deposits exposed close to the surface.
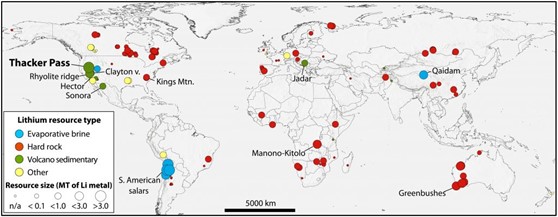
These deposits are thought to be associated with water circulation which at the right temperatures, can remove (or leach) specific elements from source rock material and deposits them in brines (e.g. evaporitic deposits, also known as salars). Lithium is a mostly fluid-mobile element, which causes it to partition into any hydrous fluid phase (instead of crystallizing mineral phases) during fluid-rock reactions and magma solidification. This elemental behavior is likely critical during formation of volcanic mudstone deposits, but no lithium deposits of this style have been commercially mined yet!
But development of commercial mining operations in volcanic mudstone deposits is probably not far off. Hydrothermal processes have been recently recognized to concentrate lithium in volcanic mudstones or clays – specifically McDermitt Caldera in the U.S. This deposit is the result of a caldera-forming volcanic eruption associated with a mantle plume – and while there is lithium present in rhyolitic magmas, there are other variables which need to align in order to concentrate lithium in economic quantities in caldera deposits.
There is substantial variability of Li content in volcanic rocks, even Li-poor source rocks appear to produce some of the largest Li-clay deposits in the world due to hydrothermal refinement within calderas (Benson et al., 2017.) However, how all these variables such as magma composition, fluid-rock interactions, post-eruptive processes, and large-scale hydrothermal systems affect the distribution of Li and associated critical minerals (e.g., REE, As, Sb, Rb, Mg) remains unstudied (e.g., Randolph-Flagg et al., 2018).
We have learned that there are 3 main styles of lithium resources. What makes things slightly more complicated is that the rocks/ geologic material associated with each style can vary in grade of lithium (i.e. amount of lithium the rocks contain), and in how difficult it is to get the lithium out of the ground and separate it from other elements (i.e. how expensive it is to extract the lithium). Let’s think about an example with a more commonly familiar metal like gold… there might be an amount of gold in the ground somewhere that’s has X monetary value, but it’s not worth mining if it’s going to cost you X amount of money (or more…) to get the gold out! This problem exists with virtually any material that needs to be extracted from the earth.
Taking lithium to the lab
Okay so we have lithium in our rocks, but now what? Well, it’s no surprise that our typical goal with geochemistry is to precisely quantify various elements and isotopic concentrations within some geological material. The elemental behavior and isotope ratio signatures of lithium can provide us with insight into hydrologic / hydrothermal processes, weathering rates, crustal recycling, and eruption timescales and dynamics.
Lithium is a very fast diffusing element as well, and this can be applied to help interpret diffusion timelines and cooling rates related to post-emplacement processes of large volcanic deposits (Ellis et al., 2015). The history behind lithium measurements is fascinating, but there are plenty of continuing hiccups and challenges… stay tuned for part two!
That’s it for the first part of this blog, part two is coming soon. If you’ve got any thoughts, questions or comments about lithium and the measurement of its isotopes, please let me know, I’d be keen to hear (emily.cahoon@isotopx.com).